Cellular Traffic Jams
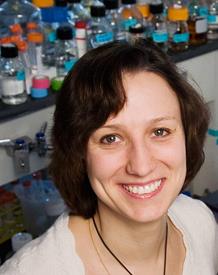
The inside of a living cell contains microscopic traffic jams that would rival the Los Angeles interstate system in congestion. And among the busiest cellular highways are the strands of DNA, which contain the blueprints for life.
Maria Spies, an LAS biochemist, is tracking the movement of traffic along this nanoscale highway, focusing on vital molecular machines known as helicases. Her team has found that some helicases have a remarkable ability to move around barriers in their path, something LA commuters would love to do. Understanding how these biological machines operate can unlock secrets to various diseases, including cancer and premature aging.
There are hundreds of types of helicases in humans, but Spies is studying the behavior of a type that plays an important role in DNA repair. When these helicases are defective, the DNA repair system can break down, triggering mutations and disease. For example, defects in the FANCJ helicase can lead to an early onset of breast and ovarian cancer; defects have been linked as well to Fanconi Anemia, which can lead to bone marrow failure, physical abnormalities, and increased risk of malignancies and other severe problems.
According to Spies, the DNA duplex—the famous two-stranded structure—“is the ideal form in which to store genetic information.” But in order to gain access to the genetic codes and make repairs, the two DNA strands have to be separated. This is where helicases come into play. As they race along the DNA, helicases break bonds between the strands, unzipping the helix and making it possible for genetic information to be accessed and DNA to be repaired.
Healthy cells continuously repair their DNA, but so do cancerous cells as they try to undo the damage done by radiation or chemotherapy. Therefore, understanding the role of helicases as cancer cells repair their DNA will help in the development of more effective treatments and will decrease side effects of radiation and chemotherapy.
Helicases were discovered in the 1970s, and until recently textbook illustrations showed a wedge-shaped molecule flying in, unwinding the DNA duplex, and then flying away.
“But we came to realize that not everything is that simple,” Spies says. “We’re interested in knowing how helicases with very similar motor cores can perform completely different tasks. Some can be extremely fast and some can be very slow when necessary. Some move continuously for a long distance along DNA, while others do not.”
In addition, some helicases can do multiple jobs, unwinding the DNA duplex and doing actual maintenance on the DNA as well. “We want to know how the same helicase can do both things,” she says. “Very different biophysical properties are required for both tasks.”
For example, another helicase that the Spies lab studies, Rtel, is important for controlling accurate DNA repair and is also involved in maintaining telomeres—protein-DNA structures found at the ends of chromosomes, protecting them from deterioration.
“We don’t know yet how it works,” she says. “But we will.”