Theory sorts order from chaos in complex quantum systems
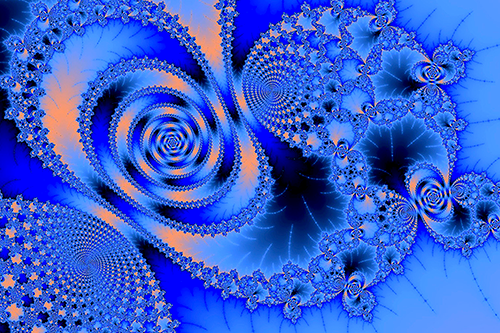
It’s not easy to make sense of quantum-scale motion, but a new mathematical theory could help, providing insight into the various computing, electrochemical, and biological systems.
Chenghao Zhang, a physics graduate student at the University of Illinois Urbana-Champaign, and chemistry professor Martin Gruebele performed a computational analysis of the new mathematical theory developed by Rice University theorist Peter Wolynes and theoretical chemist David Logan at Oxford University. The theory gives a simple prediction for the threshold at which large quantum systems switch from orderly motion like a clock to random, erratic motion like asteroids moving around in the early solar system.
In the new study, Zhang and Gruebele used computational modeling to show that the Wolynes-Logan theory predicts the nature of motions in a chlorophyll molecule when it absorbs energy from sunlight.
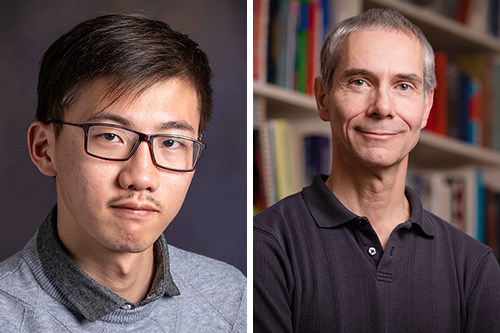
The findings are published in the Proceedings of the National Academy of Sciences.
“In thinking about the motions of individual molecules at the quantum scale, there is often this comparison to the way we think of the solar system,” Wolynes said. “You learn that there are eight planets in our solar system, each with a well-defined orbit. But in fact, the orbits interact with each other. Nevertheless, the orbits are very predictable. You can go to a planetarium, and they’ll show you what the sky looked like 2,000 years ago. A lot of the motions of the atoms in molecules are exactly that regular or clocklike.”
When Wolynes and Logan first posed the question of predicting the regularity or randomness of quantum motion, they tested their math against observations of vibrational motions in individual molecules.
Things are more complicated when the molecules also dramatically change structure, the researchers said – for instance, as a result of a chemical reaction.
“As soon as we start looking at molecules that chemically react or rearrange their structure, we know that there’s at least some element of unpredictability or randomness in the process because, even in classical terms, the reaction either happens or it doesn’t happen,” Wolynes said. “When we try to understand how chemical changes occur, there’s this question: Is the overall motion more clocklike or is it more irregular?”
Predicting at which point the transfer of energy that takes place during photosynthesis turns from orderly motion to randomness or dissipation would take a significant amount of time and effort by direct computation, the researchers said.
“It is very nice that we have a very simple formula that determines when this happens,” Gruebele said. “That’s something we just didn’t have before, and figuring it out required very lengthy calculations.”
The researchers said the Logan-Wolynes theory opens up a wide array of scientific inquiries ranging from the theoretical exploration of the fundamentals of quantum mechanics to practical applications.
“The Logan-Wolynes theory did pretty well in terms of telling you at roughly what energy input you’d get a change in quantum-system behavior,” Wolynes said. “But one of the interesting things that Zhang and Gruebele found is that there are these exceptions that stand out from all the possible orbiting patterns you might have. Occasionally there are a few stragglers where simple motions persist for long times and don’t seem to get randomized. One of the questions we’re going to pursue in the future is how much that persistent regularity is actually influencing processes like photosynthesis.”
Beyond photosynthesis, the researchers said the theory applies to any sufficiently complex quantum systems and may give insights into building better quantum computers. For instance, it could help design features of next-generation solar cells or perhaps make batteries last longer.
The James R. Eiszner Chair in Chemistry and Physics at Illinois, the Bullard-Welch Chair at Rice and the National Science Foundation supported the research.
Gruebele also is affiliated with physics, the Center for Advanced Study, the Beckman Institute for Advanced Science and Technology, and the Carle Illinois College of Medicine at Illinois.